As the realities of COVID 19 slowly dawns on us, it is becoming clear that its effect on the healthcare systems will go far beyond the disease that it produces. We would be naïve to believe that once the COVID 19 scepter recedes, the “genie has gone back in the bottle”.
Many healthcare facilities have discovered to their dismay that their existing HVAC facilities, which were ignored all along, are not only deficient for the pandemic, but are faulty or in poor condition for use even in normal circumstances
This article is not really about systems or how to design them. It is for getting to know the important considerations to be taken into account for proper design. Each one of these factors will need to be addressed to achieve a design for good patient outcomes and for safety of the healthcare workers.
We will cover the following aspects:
- Indoor Air Quality [IAQ]
- Relative humidity
- Temperature
- Pressure gradient
- Air distribution
- Air Changes
- Filtration
- Ultraviolet Germicidal Irradiation [UVGI]
Indoor Air Quality [IAQ] in Hospitals
The control of IAQ plays an important part in the prevention of infection in the hospital to protect the hospital staff and the patients, especially the immuno-suppressed & immuno-compromised patients who are highly susceptible to the adverse effects of various airborne chemicals and microbes.
Poor IAQ in hospital may result in outbreaks of sick hospital syndrome (SHS). The can cause headaches, fatigue, eye, skin irritations and other symptoms.
In commercial buildings and public places, the important IAQ parameters have been well identified. These include carbon dioxide (CO2), carbon monoxide (CO), nitrogen dioxide (NO2), ozone (O3), formaldehyde (CH2O), total volatile organic compounds (TVOC), respirable suspended particulates (RSP) and total bacterial count. These can adversely affect our health with various degrees of severity. They can result in sick building syndrome (SBS) or building-related illnesses (BRI), such as pneumonitis and cancers.
In addition to the above-mentioned airborne pollutants, there are some toxic chemicals that are used in hospitals and these can be found in the indoor space. Chemical contaminants that are of great concern for hospitals include glutaraldehyde (C5H8O2), nitrous oxide (N2O), and latex allergens.
- Glutaraldehyde is a toxic chemical. It is used as a cold sterilant to disinfect and clean heat-sensitive medical, surgical and dental equipment and equipment used in endoscopy. This chemical can cause skin irritation and large exposure can result in nausea, headache, and shortness of breath.
- Nitrous oxide is used widely in anesthesia. Exposure to this chemical can cause dizziness, fatigue, headache, excessive sweating or shivering, , nausea, or vomiting
- Latex allergens are released while wearing surgical and exam gloves or installing catheters and other tubing. Latex allergy can result in skin or mucous membranes (eyes, mouth, nose, or other moist areas) reacting when in contact with latex. A severe latex allergy can affect breathing and other serious problems.
Many of the pathogenic bacteria in hospitals are very small and range in size from less than 1micron to 5micron and are respirable. These can remain suspended in the air for a long time and this enables the disease to be transmitted easily. Some of these are mycobacterium tuberculosis (TB) bacteria, legionella bacteria, methicillin-resistant staphylococcus aureus, and aspergillus spores.
- TB bacteria: when an active TB patient coughs, sneezes, or speaks, airborne TB droplets will be generated. These droplets, if respired by others can lead to their being infected.
- Legionella bacteria: A common source of this bacterium in hospitals is the water mist plume discharged from the cooling towers and then drawn into the indoor environment through the outdoor air intake. Other sources could be evaporative condensers, potable water systems, and hot water systems.
- Staphylococcus aureus: These bacteria are present on the skin and in the nose, blood, and urine of an infected patient. During some surgical procedures that require the use of power tools, such as oscillating bone saws and bone drills, microbial aerosols will be generated.
- Aspergillus spores: Hospital renovation or nearby construction work are major sources of aerosolized Aspergillus spores. The fungal spores from soil, plants, animals, and dust particles can attach themselves to the clothing of healthcare workers or visitors (Michael Leung & Alan H.S. Chan, Control and management of hospital indoor air quality).
Relative Humidity
Relative humidity affects human comfort, respiratory system and the healthcare spaces in a variety of ways.
How humidity affects comfort
The temperature boundaries of the comfort zone are well defined in terms of laboratory and field observations. However, how humidity affects comfort is less certain, particularly the upper humidity levels.
Low humidity leads to complaints about dry-nose; throat, eyes and skin. Low humidity can lead to drying of the skin and mucous membrane surfaces leading to increasing susceptibility to respiratory disease as well as discomfort.
High humidity levels reduce comfort. It leads to sweating. Prolonged sweating leads to accumulation of salts on the skin which in turn leads to discomfort. With high humidity, clothes pick up moisture. This causes increased friction between skin and fibres and creates uncomfortable sensation.
How humidity affects the respiratory system
The normal human body has excellent protection systems to prevent respiratory infections. There are several layers of filters starting with the mouth and the wind pipe. The moist surfaces having mucous layer collect the larger particles before they enter the trachea and pharynx in the upper respiratory tract. In the lower respiratory tract, the bronchi & alveoli can trap smaller particles to various degrees of efficiency.
Moisture in the air is the first arm of our immune system and we now know that our body cannot fight off foreign particles or invaders as adequately when we’re in a dry environment. Further, the infectivity of the bacteria too increases with low humidity. Relative humidity of at least 40% is considered the threshold. (Dr. Stephanie Taylor, Presenter, “Optimize Occupant Health, Building Energy Performance and Revenue through Indoor-Air Hydration, ASHRAE).
All microorganisms need water to grow initially. Too much humidity leads to higher levels of dust mites and fungi, two of the worst culprits for indoor allergy sufferers. Mold and fungi are known to exacerbate respiratory conditions such as upper respiratory (nasal and throat) symptoms, cough, wheeze and asthma. Immuno-suppressed or Immuno- compromised patients are at increased risk.
Effect of Humidity on Airborne Transmission of Cough Aerosol
Small particles get generated during the course of coughing and sneezing and to a lesser extent by even talking and breathing. Particle size distributions of coughed materials encompass a broad spectrum of diameters, from very small to large droplets.it is known that most of the viable particles ranging from 0.65 to 3.3μm in the cough-generated aerosols are immediately respirable (K. P. Fennelly et. al “Cough-generated Aerosols of Mycobacterium tuberculosis: A New Method to Study Infectiousness,” American Journal of Respiratory and Critical Care Medicine).
While big particles and droplets from cough promptly fall to ground and surfaces, the smaller ones float in the air for a long time. Small particles <5micron (droplet nuclei or residue) are formed from droplets (usually within milliseconds) in the air which shrink in size due to the process of evaporation and desiccation in low humidity and remain suspended in air for several hours.
How humidity affects the virulence of bacteria
Organisms that have been exposed to dry environment have increased virulence and increased rates of antibiotic resistance even in the absence of antibiotics. It has been observed that bacteria that have become resistant to medication can pass that genetic material back and forth when they’re in the airborne state. In a low humidity environment in a hospital, when cough droplets containing bacteria become airborne, those bacteria can share their antibiotic resistant genes while in the airborne state. This is known as horizontal gene transfer and it is very rapid.
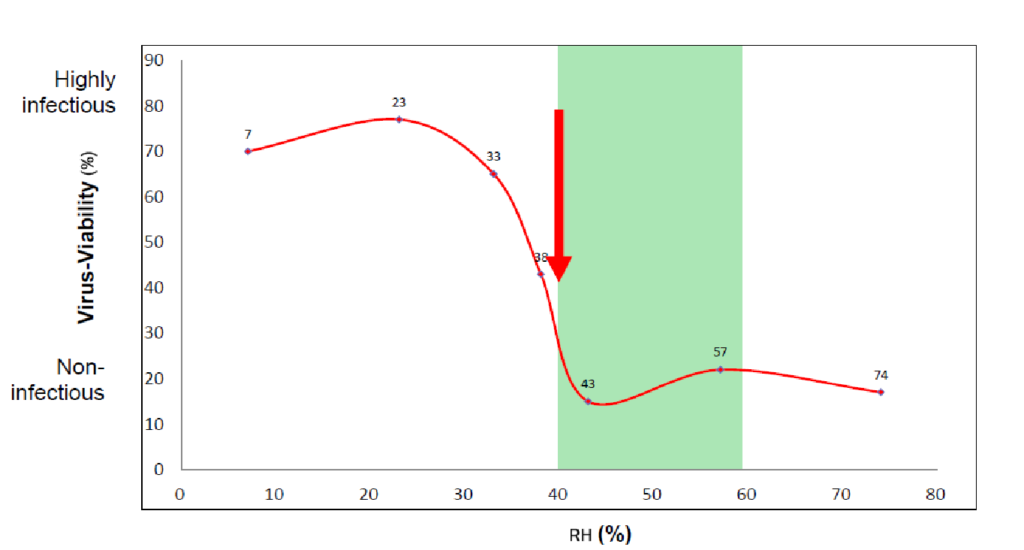
Figure 1: Stephanie Taylor, Presenter, “Optimize Occupant Health, Building Energy Performance and Revenue through Indoor-Air Hydration,” ASHRAE
Apart from virus, there is a correlation of humidity with the growth of various pathogens as given in the Sterling chart below [figure 2]
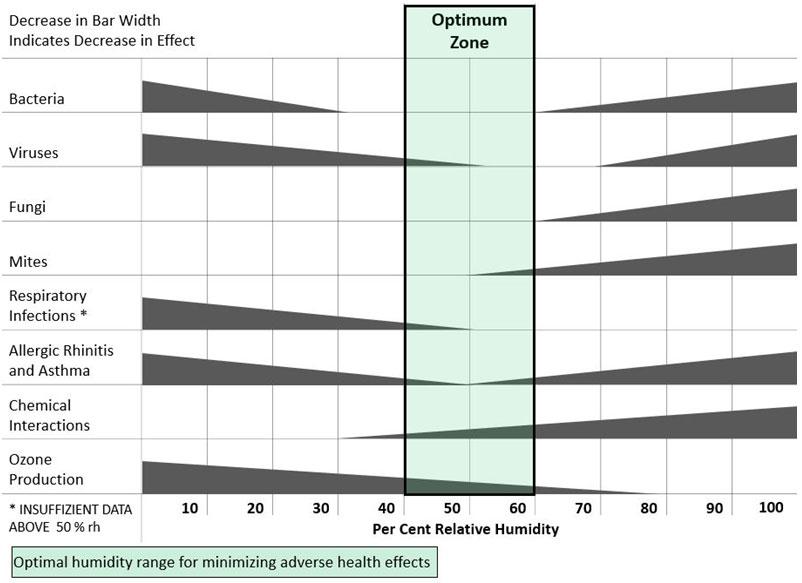
Figure 2: Criteria for Human Exposure to Humidity in Occupied Buildings, E.M. Sterling, 1985 ASHRAE Transactions CH85-13-1
All things considered, the humidity level of 40% ~ 60% is considered to be the most suitable environment for humans & decreases problems from pathogens.
How Humidity affects the hospital building
Apart from the human beings, the building habitat too is not wholesome when there is high humidity. Of all the conditions needed for the growth of mold, moisture is the simplest and most universal requirement for fungal growth. Preventing moisture from getting into the food sources is a good way to prevent fungal growth from becoming established. Actual condensation of moisture leads to the growth of some of the most hazardous species of mold. However, there are thousands of varieties of fungi that do not require liquid water to germinate. When humidity rises every day, the air slowly permeates moisture into the food sources. When moisture content and temperature remain high for long periods of time periods when the air conditioning is turned off, the extra moisture needed to support fungus is gets added to paper, organic materials and other food sources in the rooms.
In addition to creating health problems, moisture can damage the hospital building materials and components. Some examples are given below:
- Wooden materials, varnishes and paints get damaged and adhesives fail.
- Insulations deteriorate and the thermal coefficient of resistance reduces as moisture pervades the insulation material cells of the space between materials.
- Anchor fasteners and structural members, air conditioning ducts corrode.
- Prolonged damp conditions can lead to mold and bacteria and insect pests colonizing the building material.
It is thus important that there is humidity control in hospitals to protect the patients and other inhabitants as well as to protect the hospital building itself.
Temperature
The patient’s thermal wellbeing is largely a function of temperature, not humidity. This fact alone makes temperature a very important parameter. The normal comfort temperature range for patient rooms is considered 21~24C. Depending on the clothes worn and the air velocity, ISHRAE IEQ standards indicate the people can be comfortable between 24C to 30C.
Patients in both clinical & inpatient facilities may be very scantily dressed or without clothes and sometimes for long periods of time. The temperatures should be controllable so that the patient can have higher temperature for comfort.
In a variety of disease or injury, patient metabolism or fever can interfere with body’s ability to regulate heat. Burn units in general will be required to have capability of heating the space to 32.2C.
For operation theatres & emergency department healthcare workers often wear layers of clothing mostly un-permeable and will feel very hot inside. They may require temperatures as low as18C.
The HVAC Design Manual for Hospitals and Clinics [ASHRAE] gives the following chart which includes special temperature requirements for Operation Theatres:
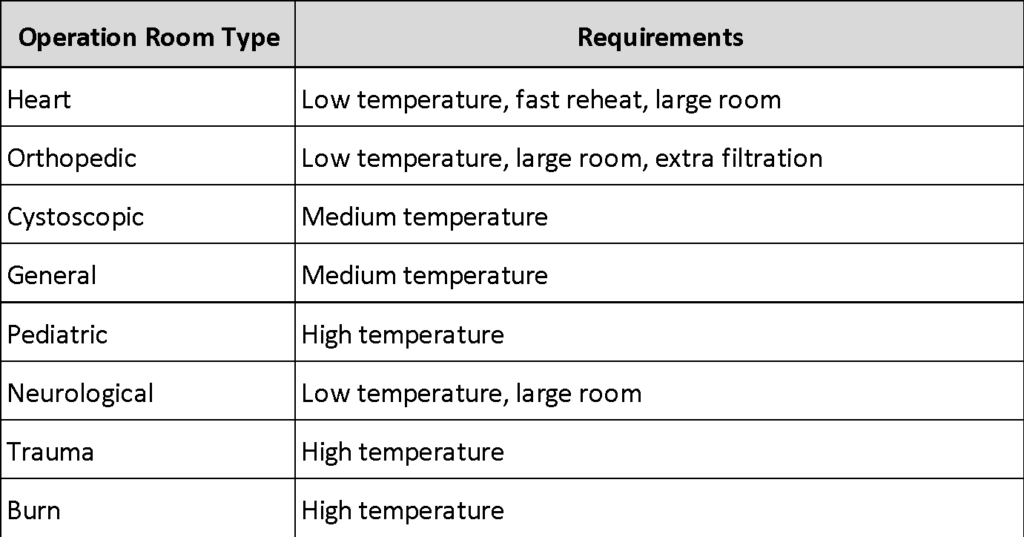
Pressurization
While the quantity of air distributed to the space is important, from an air-quality standpoint, space pressurization is perhaps even more important. The air quantities of supply, return, fresh air and exhaust should be balanced so that air travels from the cleanest spaces (such as operating rooms) to the dirtiest spaces (such as soiled utility or decontamination rooms).
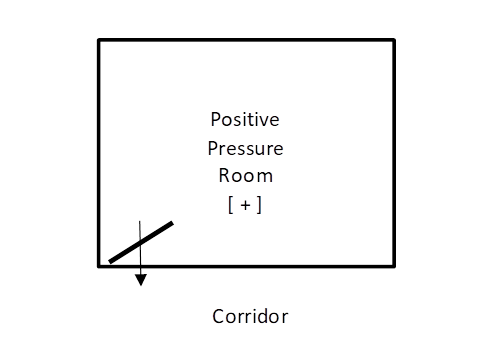
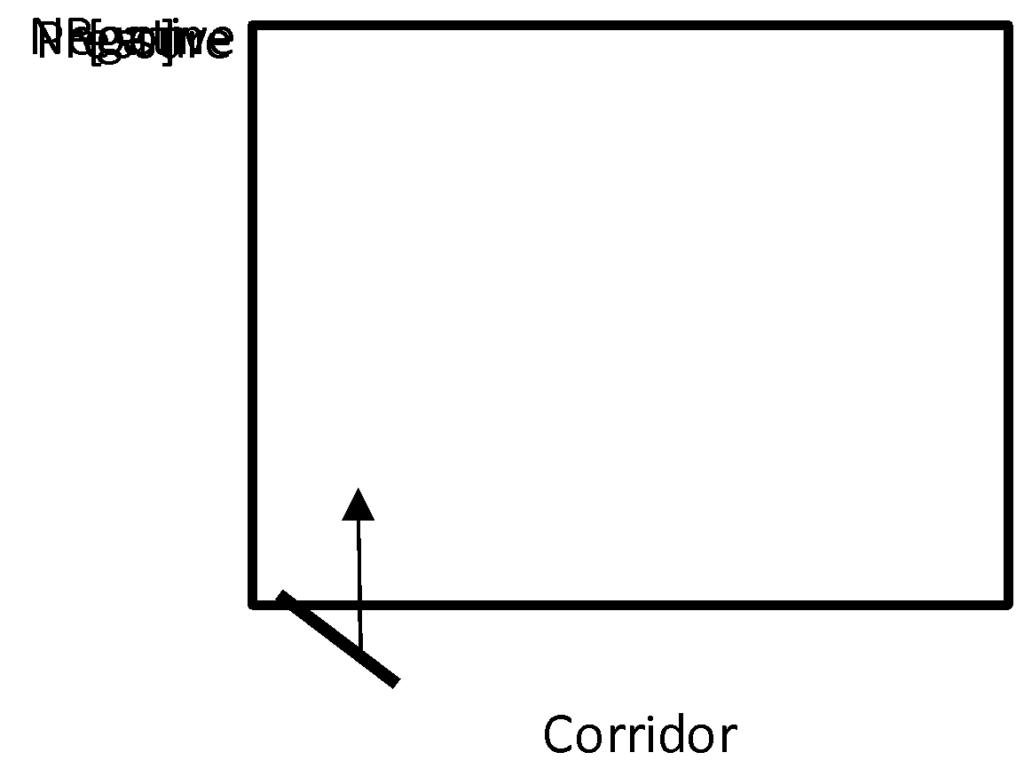
In healthcare facilities, the convention is to have the differential pressure to be least 2.5 Pa so that any interference due to door opening, elevator movement and other normal activities will not change the positive or negative nature of the pressure or the airflow direction.
The pressure differentials recommended for the isolation rooms are as follows as per the CDC are: Protective Environment Isolation Rooms [P.E] (Positive Pressure Rooms): Minimum 2.5Pa & ideally >8Pa Airborne Infectious Isolation Rooms (A.I.I.) Rooms: Minimum > – 2.5Pa
A pressure differential of 2.5 Pa is recommended by NABH for Operation Theatres. Such a low pressure is difficult to monitor and it would be prudent to design and maintain the differential pressure at >8Pa.
The ASHRAE Standard 170-2017 has a recommendation for the pressure gradient for the various rooms in a hospital which indicates whether they should be positive, negative or neutral with respect to the surroundings.
It is very important to understand that in addition to the pressure differential, which can force a desired airflow path carrying particles, the particle concentration differential can also create an air exchange due to mass diffusion between two areas with a significant particle concentration difference to allow the concentrations in both rooms to reach equilibrium.
This means that, even if there is a pressure differential between adjoining areas separated by a door, if the less clean area is significantly dirty, there will be a mass diffusion of particles from the dirty area into the clean area in spite of the latter area being at a higher pressure.
The risk level of particle contamination can be expressed as contamination rate (CR) (Sun et al.2011). CR is defined as the airborne particle concentration gain above the initial concentration in a clean space over the particle concentration in the adjoining less clean space that is the source of the contamination. CR is a criterion to quantify the effectiveness of a cleanroom barrier in preventing particle migration into the clean space. The lower the CR level, the better the performance of the barrier’s effectiveness. A typical contamination ratio study is given below.
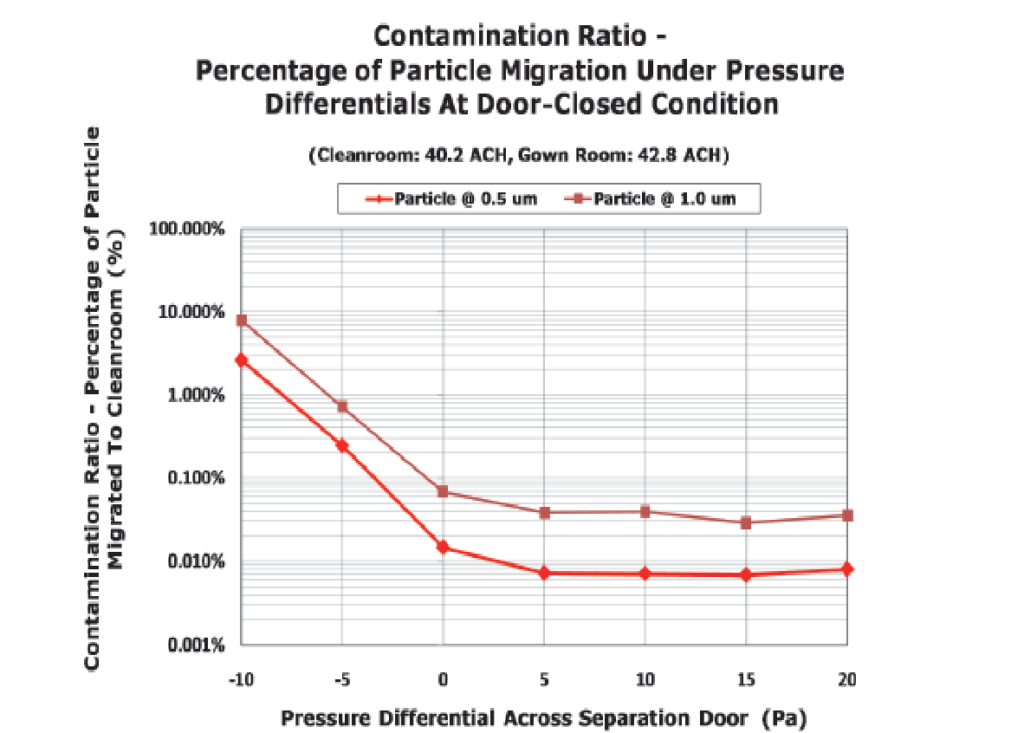
It is thus important that clean spaces open into adjacent areas which are not significantly less clean.
Introduction of an airlock (also called ante room) outside a clean space to be protected can significantly improve the barrier effectiveness. This illustration from ASHRAE Design Guide for Cleanrooms makes the concept clear.
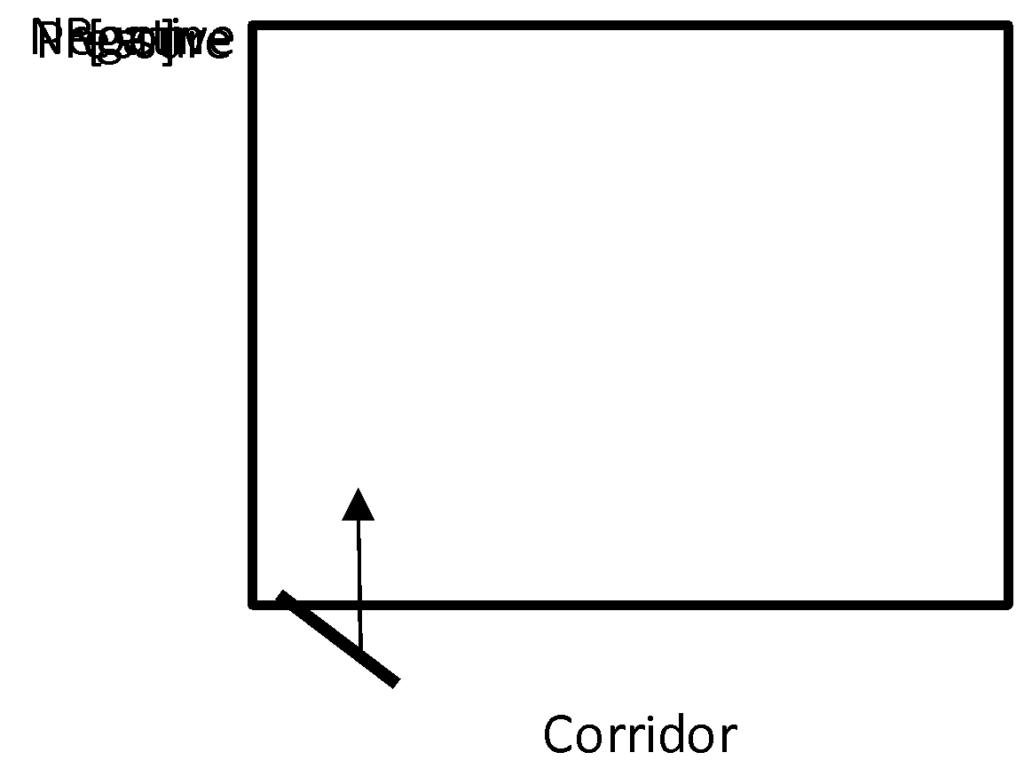
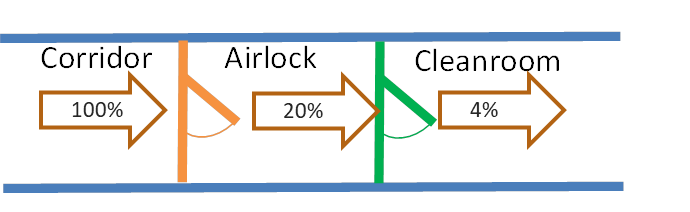
Figure 7: Barrier Effectiveness of Airlocks [[ASHRAE Design Guide for Cleanrooms].
It is common to have a clean corridor acting as an airlock for a number of operation theatres. Especially for negative isolation rooms, airlock is recommended. The use of airlock enhances the contamination barrier protection offered by pressure differentials.
When there is an airlock, the two doors are interlocked so that both the doors do not open simultaneously.
For pressurization it is important that the rooms should be of tight construction and the doors should be of good quality with minimum possible gap between the door frame and the door gasket.
It should be noted that when a door is of the swing type, there is a piston effect on the air when it is opened. This effect brings in its wake a surge of outside contaminated air into the clean space. Especially for operation theatres it is a good idea to have sliding doors as opening and closing these doors does not create the piston effect.
Air Distribution
For infection control, it is not only about air filtration and differential pressure. How the air is introduced into the room and then how it sweeps way the contaminants is important. A few examples will make the concept clear.
- In an Airborne Infectious Isolation [A.I.I.] room, the people in the outside corridor and the healthcare workers will need to be protected from the patient. The room is thus kept under negative pressure. The healthcare worker should be safe when attending to the patient and the latter’s respiration should be directly exhausted. The air pattern shown in the figure does just that. The clean air from the supply air duct comes first on the healthcare worker & thence to the patient and then exhausted from the nearby exhaust grille.
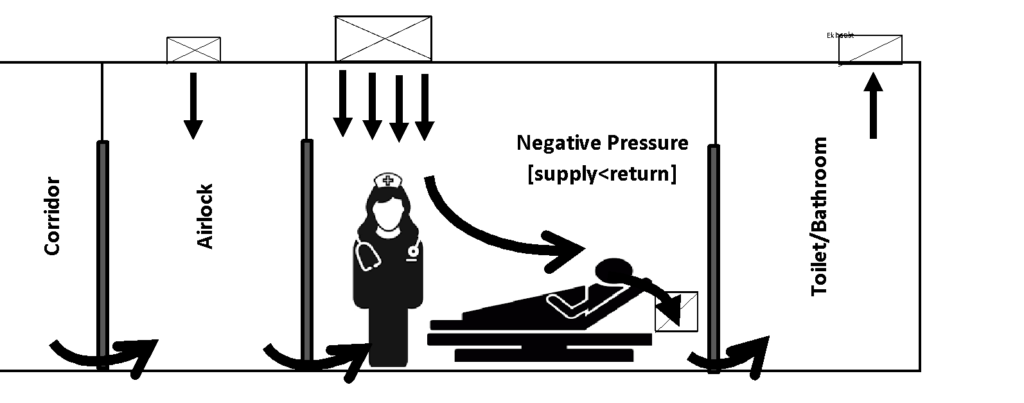
Figure 8: Air Pattern for a negative pressure A.I.I. Room
- In a Protective Environment Isolation [P.E.] room, the people in the outside corridor and the healthcare workers should not infect the patient, who is immuno-compromised or immuno-suppressed. The room is thus kept under positive pressure. The healthcare worker’s respiration when attending to the patient should be directly exhausted. The air pattern shown in the figure tries to achieve this. The clean air from the supply air duct comes first on the patient & thence to healthcare worker and then exhausted from the nearby exhaust grille.
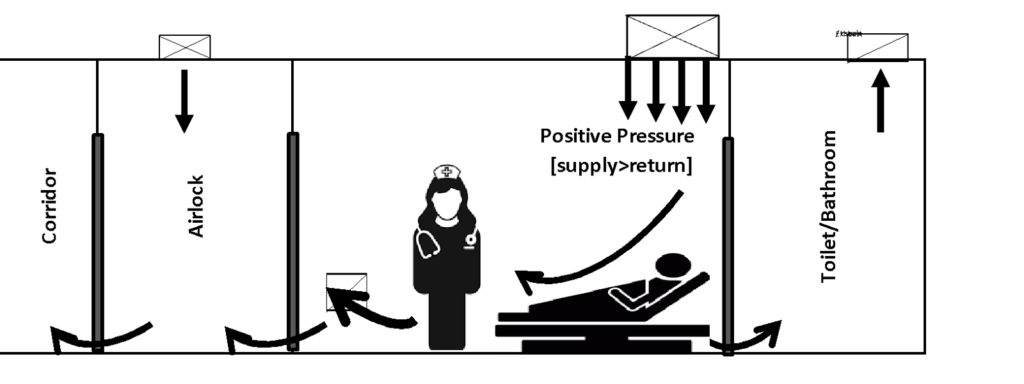
Figure 9: Air Pattern for a positive pressure P.E. Room
- In an operation theatre [OT] the room is kept under positive pressure to protect the space from the less clean outside corridor or airlock. Inside the OT, there are a lot of contaminants that get released from the surgical procedures. The unidirectional airflow from the HEPA filters covering the patient and the surgical staff provides clean air in operating table zone which includes the patient as well as the surgical staff. The key to prevent surgical site infection is to sweep the contaminants released away from the wound and into the return air risers.
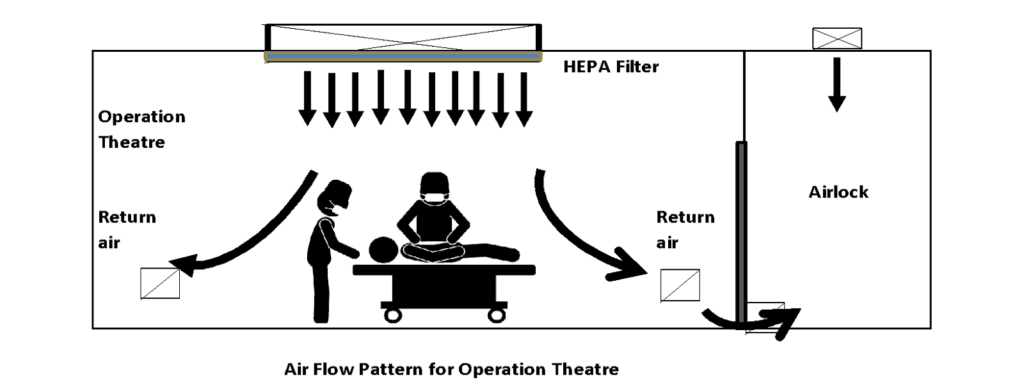
Figure 10: Air Pattern for an Operation Theatre
Air Flow Patterns in Other Areas
The air patterns in rooms dealing with emergency waiting rooms, nuclear medicine, chemical decontamination rooms and TB patient waiting rooms are important and need to be a part of design deliberations as this will impact transmission of disease.
Air Change Rates
Air change rate represents the number of times the entire volume of air in the room is replaced each hour.
One of the methods to reduce the exposure of the person to the concentration of toxic chemicals and the number of microbes is by providing ventilation i.e. introducing clean fresh air into the area. More number of air changes of ventilation per hour reduces the exposure of the patients and the healthcare workers to the toxic chemicals and microbes.
Similarly, certain quantity of air is recirculated in the space through the air filtration system of the air handling unit. The number of times the air gets churned through the filtration system results in more of the particulate matter and the microbes getting filtered out of the air. This recirculation of air is also expressed in air changes per hour.
The chart given below is from the CDC and it shows the time required for airborne-contaminant removal efficiencies of 99% and 99.9% through air change rates. The chart assumes perfect mixing of the air within the space (i.e., mixing factor = 1). However, perfect mixing usually does not occur. Removal times will be longer in rooms or areas with imperfect mixing or air stagnation.
Air changes/hour (ACH) and time required for airborne-contaminant removal efficiencies of 99% and 99.9%*
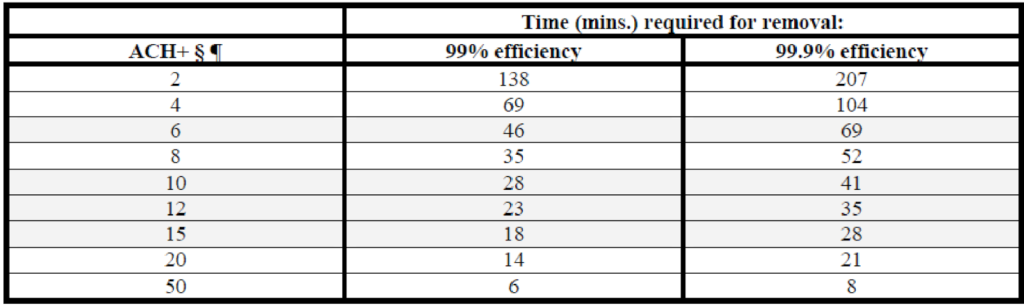
Figure 11: Air Change Efficiency Chart (CDC)
§ Values were derived from the formula:

A typical air change rate of 12ACH would indicate that the microbes generated in the room will be resident in the room for 23minutes before being removed.
For the OTs NABH requires a minimum of 20ACH of air recirculation and 4ACH of outside air make up.
As per ASHRAE Standard 170-2017, some of the spaces have the following outside air and recirculation requirement:
Filtration
Air filtration has huge role to play to protect humans in healthcare facilities whether it is hospitals, out-patient facilities, doctor’s office or clinics. In many of these places the occupants are already immuno-compromised or immuno- suppressed and are thus more susceptible to airborne contaminants than normal healthy people.
Filters can effectively trap particulate contaminants, including microbiological pathogens, and remove them from the circulating air. Various grades of filters can be used to achieve different degrees of cleanliness. For a healthcare facility, a proper filtration system generally consists of a pre-filter and a final filter in the air handling unit (AHU). The pre-filter should be placed upstream, ahead of the cooling/ heating coil, to remove large particles for a clean heat transfer medium. More importantly, the pre-filter can prolong the life of the final filter placed downstream of the AHU, resulting in a cost-effective operation.
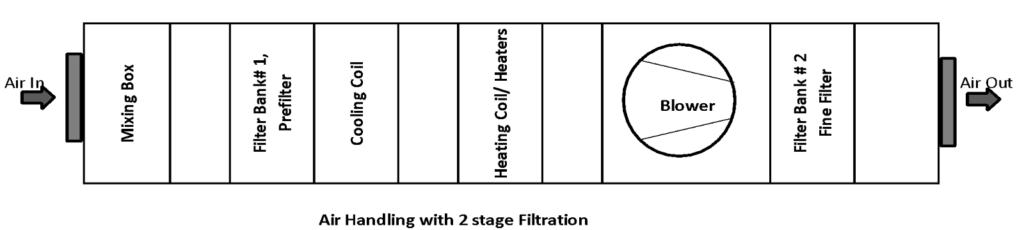
Figure 12: Two Stage Filtration in an Air Handling Unit
Filter Classifications
The ASHRAE Standard 52.2 classifies filters used in HVAC application by their Minimum Efficiency Reporting values (MERV). The standard takes into consideration the fractional efficiencies of filters for particles in the size range of 0.3 to 10 microns. The test not only provides the initial efficiencies, but also the minimum efficiencies for different particle sizes in a loading test. Filters are given their MERV numbers based on their “Average minimum particle size efficiencies” for the three size range groups, namely E1 for 0.3 to 1 microns, E2 for 1 to 3 microns and E3 for 3 to 10microns. For Coarse filters, the standard also provides a methodology for measuring the Gravimetric Arrestance.
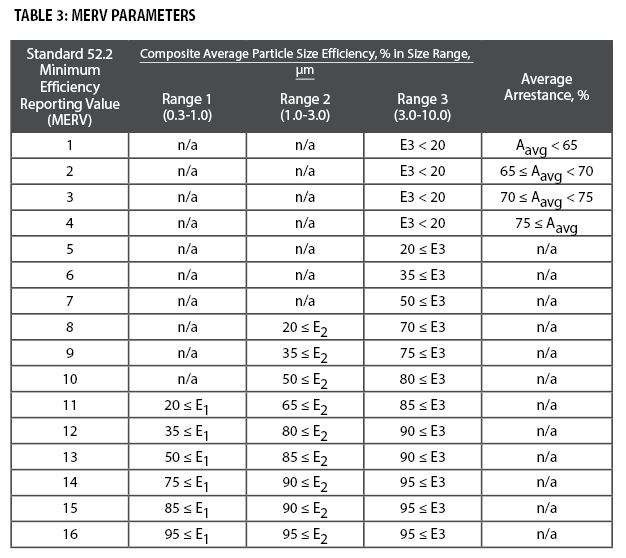
Figure 13: Minimum Efficiency Reporting Values (MERVs) and Filter Efficiencies by Particle Size
Air filters of MERV 13 and above have the potential to remove microbes and other particles ranging from 0.3μ to 10.0 μm.
HEPA Filters
High efficiency particulate air (HEPA) filters trap a vast majority of very small particulate contaminants from an air stream.
The three main mechanisms by which HEPA filters achieve filtration are: interception, inertial impaction and diffusion.
Particles less than 0.1 micrometers are easily trapped due to diffusion while particles larger than 0.4 micrometers are trapped by inertial impaction and interception. Particles between 0.1 and 0.4μm are therefore too large for effective diffusion and too small for inertial impaction and efficient interception. So, the filter’s efficiency drops within this range and the filter exhibits its lowest filtration efficiency for a particular particle size. This worst case particle size is called as the Most Penetrating Particle size (MPPS), and the efficiency of a filter at MPPS is called as the MPPS Efficiency. A HEPA filter would have a overall filtration efficiency of above 99.95% at MPPS. For any other particle size, smaller or larger than the MPPS, the filtration efficiency of the HEPA filter would be greater than its MPPS efficiency. The MPPS of a HEPA filter would most commonly fall between 0.10 to 0.30 microns.
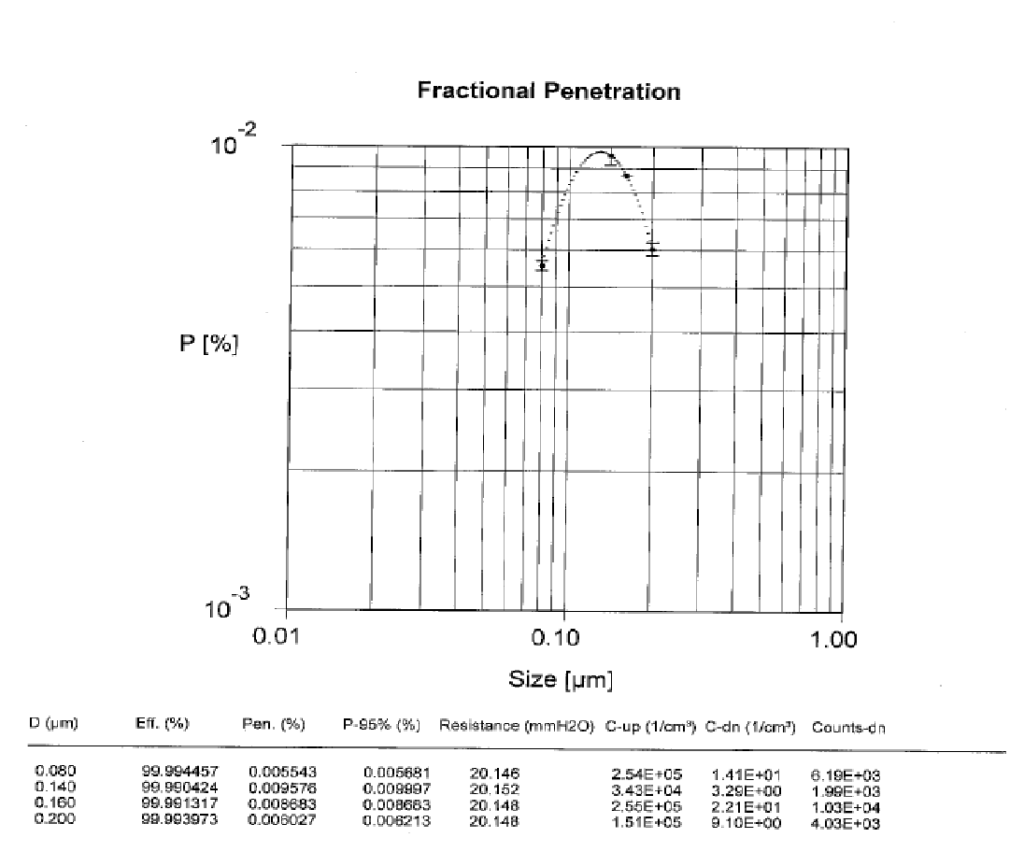
Figure 14: Typical HEPA Fractional Efficiency Test Report showing MPPS at 0.11μm
Most viruses, including CoVs, range from 0.004 to 1.0 m (Goldsmith CS et. al 2004, Ultra-structural characterization of SARS coronavirus). However, viruses are rarely observed as individual particles, but instead are expelled from the body already combined with water, proteins, salts, and other components as large droplets and aerosols. Thus far, SARS-CoV-2 has been observed in aerosolized particles in a spectrum of sizes, including 0.25 to 0.5 m (Liu Y et. al, 2020. Aerodynamic characteristics and RNA concentration of SARS-CoV-2 aerosol in Wuhan hospitals during COVID-19 outbreak) necessitating high efficiency filtration techniques to reduce the transmission potential of pathogens such as SARSCoV-2,
It can be seen from the typical performance chart of HEPA filters that viruses and bacteria can be trapped with a high degree of efficiency (>99.95%).
Ultra-low penetration air (ULPA) filters offer an efficiency of above 99.9995% at MPPS efficiency. They are used in extremely critical applications.
HEPA & ULPA filters are classified as per their filtration efficiencies at MPPS. The classification chart of ISO 29463 is given below:
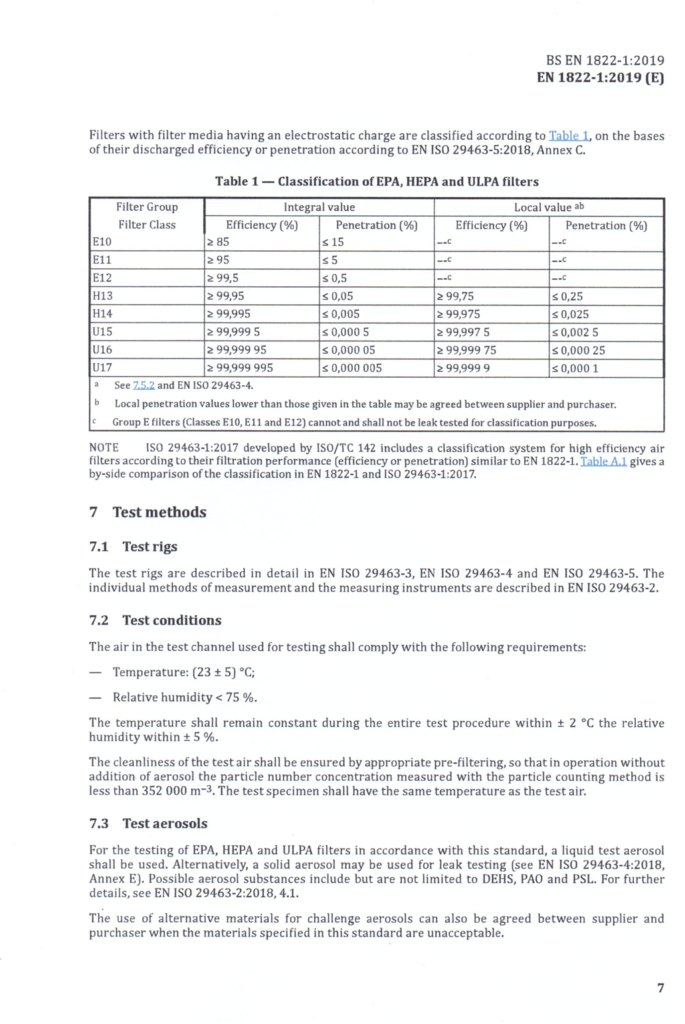
Figure 15: Classification of EPA, HEPA and ULPA Filters
HEPA# High Efficiency Particulate Air (filter) ULPA# Ultra Low Particulate Air (filter)
EPA# Efficient Particulate Air (Filter) MPPS# Most Penetrating Particle Size*
Filter Selection for Various Locations
The ANSI/ASHRAE/ASHE Standard 170-2017 gives the minimum filter efficiencies for the critical areas and this chart is reproduced here for ready reference. Depending on the actual threat perceptions higher efficiency filters may be called for.
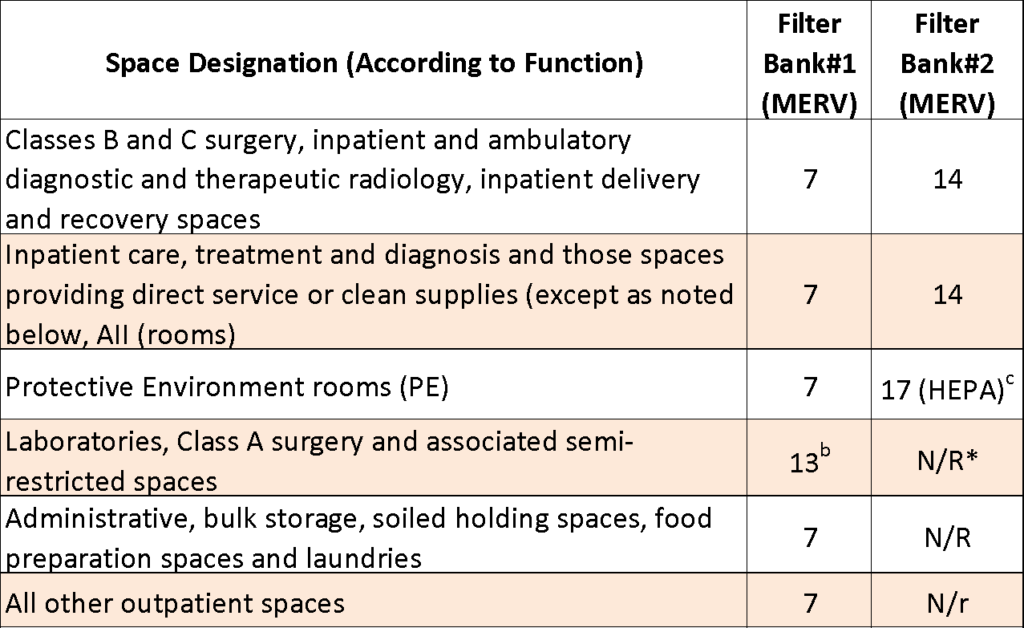
Notes:
- MERV is minimum efficiency reporting based on method of testing described in ANSI/ASHRAE 52.2
- Additional filters may be used to reduce maintenance for filters with efficiencies higher than MERV 7.
- Filter Bank # 2 may be MERV14 if MERV 17 tertiary terminal filter is provided for these spaces.
- N/R = not required.
Figure 16: Minimum filter efficiencies for the critical areas
In a critical area serving an immuno-suppressed or immuno-compromised patient, high-efficiency particulate air (HEPA) filters should be used as terminal supply air filters. In many of the operation theatres, especially for orthopedic operations, terminal HEPA filters area also used. In emergency waiting rooms where there may be infectious patients and in ICUs for infections patients, the return air would tend to have a heavy load of pathogens. In such spaces, if air is recirculated, the return air to the AHU should have HEPA filter.
How Humidity Affects Filters
An important aspect of filtration with the above methods of filtration is that, the dust, bacteria and other pathogens get collected in the filter. Some of these could act as nutrient substrate for growth of micro-organisms. High humidity can create a suitable environment for the growth of micro-organisms. Humidity levels above 85% escalate the problem. Especially when we locate the Filter Bank#2 after the cooling coil, the filters will be subject to high humidity, typically as high as 90% to 95%. With the use of higher air quantity through the air handling unit and bypassing the extra return air , which could be typically at 60% relative humidity, around the cooling coil and not passing through it, it is possible to reduce the humidity to below 85%.
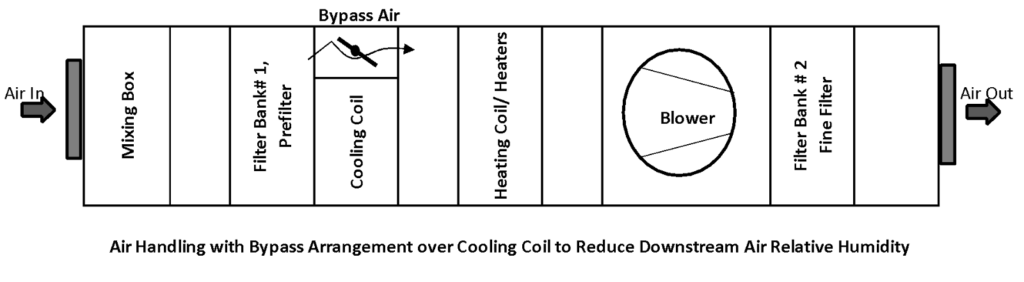
Figure 17: Cooling Coil Bypass Arrangement
Outdoor Air Intake and Filtration Requirement
The outside air quality varies greatly with the location of the healthcare facility. PM2.5 levels can be inordinately high in certain places or localities. It is recommended to use MERV13 or better grade of filters for outdoor air intake.
We have to be concerned where the outdoor air comes from as it can be a source of disease. These intakes have to be far away from cooling tower mist plumes which may carry Legionella bacteria which can cause Legionnaire’s Disease. Similarly care has to be taken that the outdoor air does not breathe in chimney exhausts from diesel generator sets, kitchen exhaust and other exhausts from the vicinity.
Air intakes are accumulation sites for dirt and debris, including rotting botanical materials like leaves, which are growth sites for fungi such as Aspergillus fumigatus. All intakes shall be designed to prevent entrainment of wind driven rain, shall have features to drain away rain water & have bird screens.[HVAC Design Manual for Hospitals and Clinics].
Room Exhausts and Filtration Requirements
Hospital exhausts can have inordinate load of pathogens and we have to be careful where we exhaust the air. The exhaust air must be protected for containment by at least MERV10 grade filters [HVAC Design Manual for Hospitals and Clinics]. Certain exhausts such as those for A.I.I. Rooms will need HEPA filters. Another way to exhaust is to take a chimney and disperse high up into the air for dispersion. This may need studies to determine that the exhausts do not harm the neighborhood.
UVGI (Ultraviolet Germicidal Irradiation)
In HVAC systems, UVGI is being increasingly used for surface sterilization (for cooling coils and condensate drain pans and as a supplement to filters for air purification.
UVC Germicidal Ultraviolet wavelength (254nm) is effective in penetrating the cell membrane breaking the DNA structure of a micro-organism. DNA sterilization inhibits reproduction. Micro-organisms such as mold, bacteria & viruses will be destroyed with the required concentrations of germicidal irradiation.
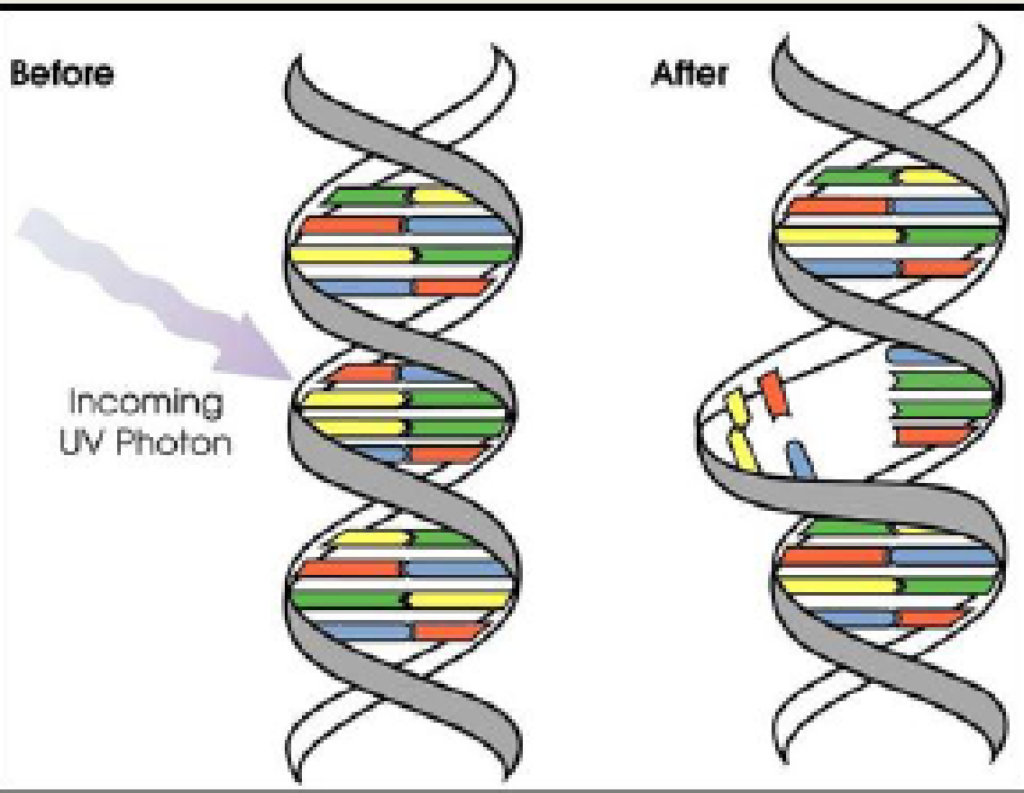
All viruses and almost all bacteria (excluding spores) are vulnerable to moderate levels of UVGI exposure, but the magnitude of the effect is species-dependent.
Out of the ultraviolet spectrum, it is the UV-C that has the maximum capability to destroy the microorganisms.
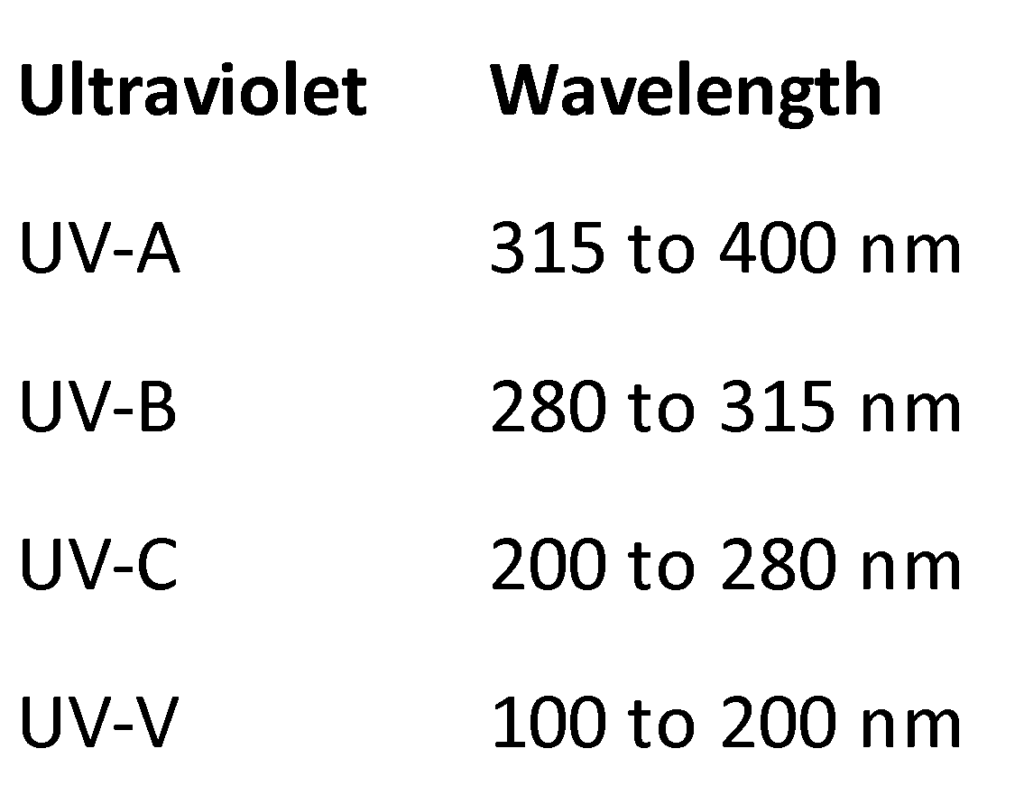
There are a number of factors to be considered that affect the deployment of UVC
- Residence time: Greater the exposure time (contact time between the contaminant and the UV source) results in more UV energy being delivered to the contaminant resulting in a greater Kill Rate.
- Intensity: Greater intensity results in more UV energy being delivered to the contaminant resulting in a greater Kill Rate
- Reflection: Reflection of the UV rays using reflectors and the inside surfaces of the AHU or duct can be a helpful tool to considerably increase the effectiveness of the UV rays produced.
- Relative Humidity: affects the susceptibility of microorganisms in the AHU as well as the duct where it is used. The susceptibility factor for the viruses was higher at 55% RH than that at 85% RH, possibly because under high RH (Tseng et al.: Inactivation of virus-containing aerosols by ultraviolet germicidal irradiation, Aerosol Sci Technol 2005). Microbial susceptibility to UVGI—may increase or decrease, depending on the organism.
- Temperature: Temperature affects the UV output of the lamp. Temperatures inside the AHU & duct can be below 13.3C [56F] and the output would be lower than 50% of the full output.
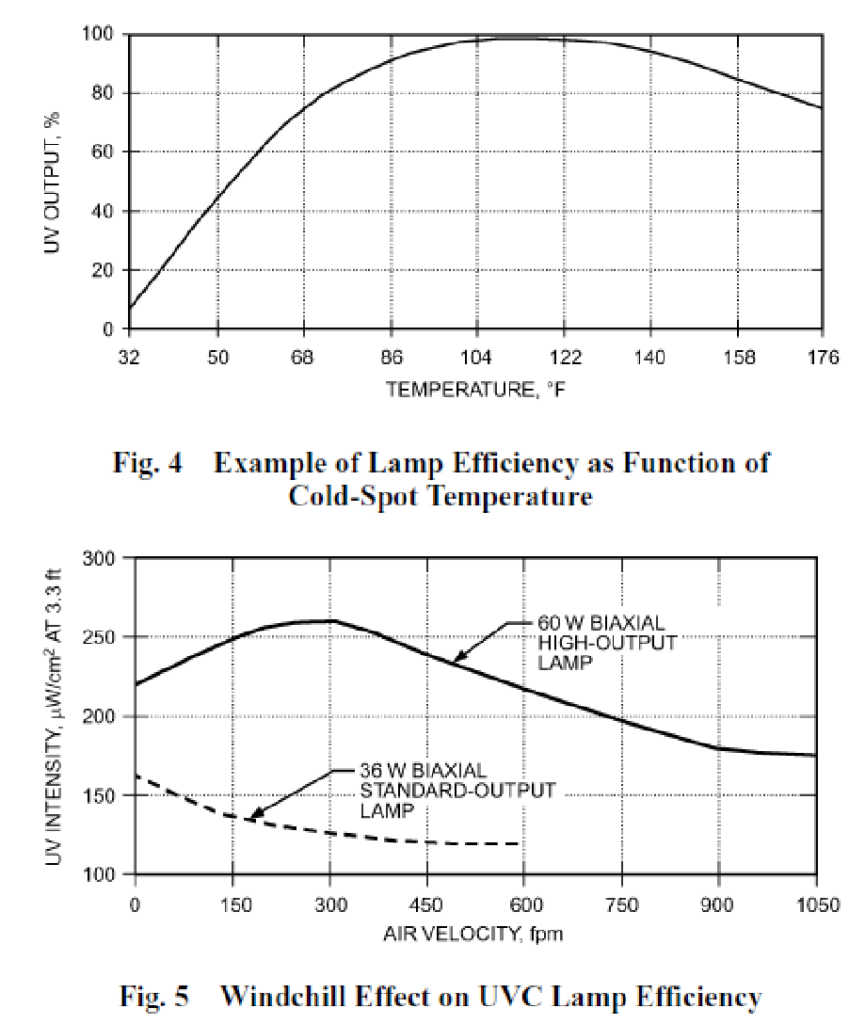
Figure 19: Output of UVGI lamps at various temperatures as a percentage of the peak output. And Wind-chill Effect on UVC Lamp Efficiency (ASHRAE 2012 Systems and Equipment Handbook, Chapter 17)
This brings us to the question about the right location of the UVGI lamp in the AHU. UV systems may be installed either Upstream (return side) or downstream (supply side) of the evaporator coil. Either installation will keep the evaporator coil clean.(2008 ASHRAE Handbook HVAC Systems & Equipment Chapter 16). The selection of the lamp wattage has to be appropriately considered.
Another consideration for the lamp selection is the lamp fouling factor. There is an advantage of locating the lamps downstream of high efficiency filters to protect them from dust accumulation. Otherwise, the lamps will need to be cleaned from time to time.
A point to note is that UVC can disinfect a pathogen only if it can reach it either directly or through reflection. In general, reducing the total number of pathogens reduces the risk of transmission.
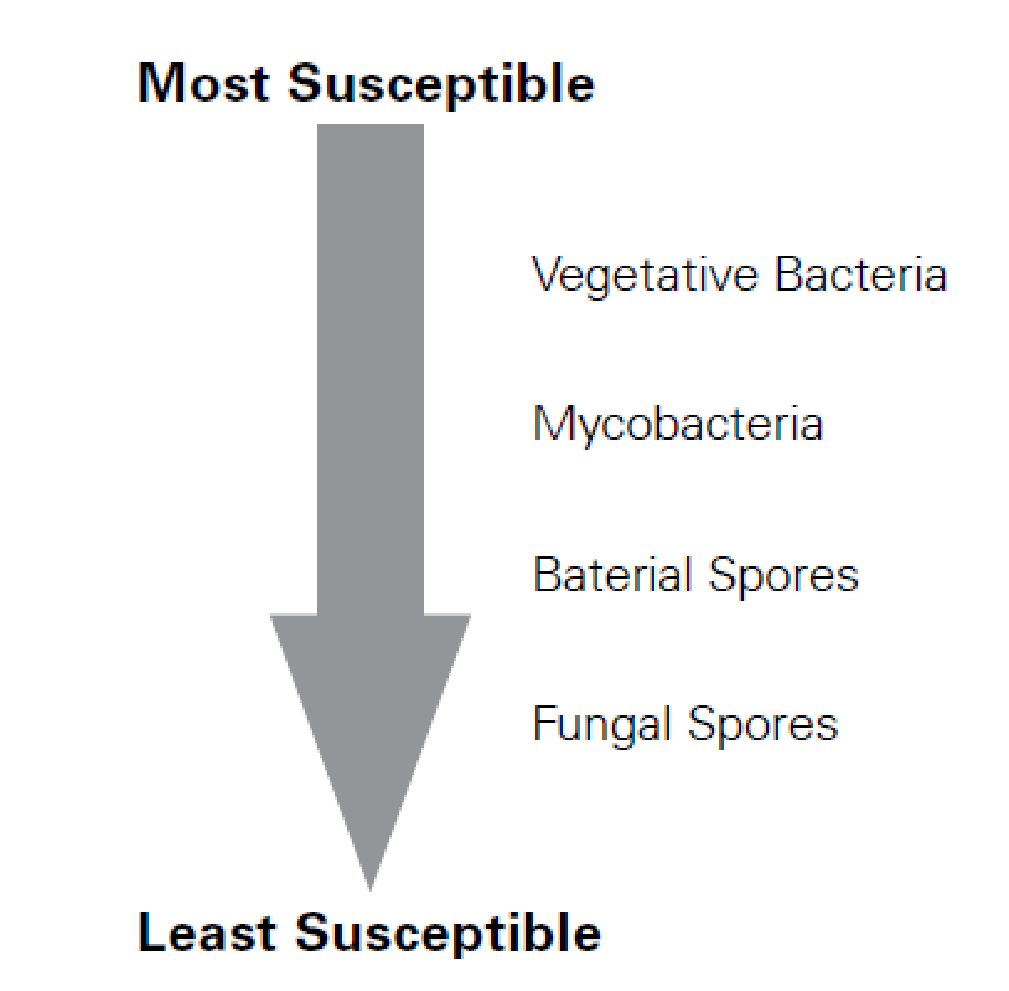
UVGI is increasingly used as the concerns about indoor air quality are growing. UVGI is being used as an engineering control to interrupt the transmission of pathogenic organisms, such as Mycobacterium tuberculosis (TB), influenza viruses, mold, and possible bioterrorism agents (Brickner 2003; CDC 2002, 2005; General Services Administration 2003).
Fungal spores are larger than most bacteria and are more resistant to UVGI than most bacteria. For spores, UV-C exposure is postulated to result in the formation of lethal photoactive products [Memarzadeh et al, American Journal of Infection Control, June 2010] For removal of spores, high efficiency air filtration, viz. MERV14 or superior, would be a better choice. Using a combination of UVGI and high efficiency filters can be an effective solution is certain situations.
UVGI is used in two formats in the air conditioning systems:
- In AHUs: UVGI directed at environmental surfaces can damage microorganisms present or growing on the surface. Lower-intensity UVGI is effective for surface inactivation because irradiation is applied continuously. UVGI from lamps in AHU plenums has been used successfully to inactivate microorganisms present on airstream surfaces such as on cooling coils and drain pans (Menzies et al. 1999, 2003). This helps in keeping the cooling coils and drain pans clean. Since the bio films that normally develop on the heat exchanger surface get eliminated, studies have indicated better heat transfer because of the UVGI radiation.
Menzies et al. found a significant decline in building-related symptoms associated with use of UVGI in AHUs though significant declines in airborne levels of fungi and bacteria as well as endotoxins were not detected in the workplace
- In Ducts: UV lamps placed in ductwork have been used to inactivate Mycobacterium species and other microorganisms. The residence time of the microbe under UVGI illumination is an important factor while considering the UVGI lamp design inside the duct. This is achieved by installing the UV lamps parallel to the airflow inside the ducts.
Safety Requirements
Inadvertent exposure to UVC can be dangerous for humans. Doors, covers and lamp mounting brackets that give direct access to Ultraviolet (UV) radiation lamp systems shall be equipped with an interlocking mechanism that removes power from the ultraviolet (UV) radiation lamp system when the door is open or cover removed.
Further, polymeric cabinet, structural, and current carrying parts and wiring which are subjected to irradiance from a UV lamp system will need to be shielded from the UVC light or be constructed of a material that is capable of withstanding UVC exposure levels expected in the product without degrading.
In Conclusion
The importance of HVAC parameters that need to be controlled have been elaborated together with the requirements of filtration. UVGI can be used as an augmentation to filtration to provide further air purification. All these have to find their place in the room design for the various areas of the facility. This has to be followed up with efficient system design and its implementation to make the healthcare facility safe for the patients, healthcare workers and the visitors.
The cost of ownership of a facility includes operation and maintenance and revalidation of the installed parameters on a regular basis. Maintaining all the air handling systems together with ducts, filters and all the equipment coming in contact with air is of paramount importance. This cannot be over emphasized.
The ultimate indicator of the success of the HVAC systems will be the patient outcomes and the low cases of HAI (Hospital Acquired Infections) and SSI (Surgical Site Infections) which are ascribed to airborne infections.
This article comes in the milieu of the COVID 19. It has exposed the malaise in the HVAC systems of many of the healthcare facilities. Some of the glaring issues that need to be addressed are:
- Meeting the ventilation requirements.
- Use of unitary re-circulatory air conditioning equipment like cassettes and Hi wall units in critical areas like ICUs and Emergency Waiting Rooms without meeting the required ventilation, filtration and pressure gradient.
- Use of false ceiling return instead of ducted return. False ceiling return will carry with it all the pathogens from the false ceiling plenum which is not accessible for cleaning.
- Inability of the infrastructure to induct extra ventilation required during pandemic.
- Inadequate isolation rooms or for that matter having a provision to convert some of the patient rooms quickly into isolation rooms to address surge in patients during pandemic.
- Engineers and technicians who have gone into a limbo due to inadequate training to handle pandemics.
All the mentioned shortcomings will need to be addressed before it is business as usual! It is sincerely believed that we will put things right in good earnest post COVID 19.